![]() |
Nov. 2024. |
News:
- Research project on direct imaging ion transport in quantum dot thin films for neuromorphic computing using XSTM is awarded by UW-Cardiff seed grant
- Research project on 2D magnetic domains is awarded by NSF, DMR program
- Research project on Reducing the Carbon Footprint of Concrete and Masonry Structures is awarded by Engineering Initiative at University of Wyoming
Recent Publication:
- Alirza Orujov, Jon M. Pikal, TeYu Chien, Jefferson A. Carter, and Saman A. Aryana, "Magnetic nanoparticle detection methods in the context of complex fluids”, Int. J. Coal Sci. Technol. 11, 86 (2024).
- Dinesh Baral, Rabindra Basnet, William R. Scougale, Raz Q. Rivlis, Dinesh Upreti, Yuri Dahnovsky, Jin Hu, and TeYu Chien, "Possible Coexistence of Magnetism and the Paramagnetic Singularity in Lightly Fe doped WTe2", Phys. Rev. B 109, 245419 (2024).
- Lauren Kim, Prince Sharma, William R. Scougale, Nozomi Shirato, Sarah Wieghold, Rose Volker, Wei Chen, Ganesh Balasubramanian, and TeYu Chien, "Distinguishing Elements at the sub-nanometer Scale on the Surface of a High Entropy Alloy", Adv. Mater. 36, 2402442 (2024)
Toward Understanding Novel Materials' Properties
In our lab, we devoted our efforts to study the physical properties of materials, especially the properties of electrons, phonons (vibrational modes) and their interactions. Our goal is to provide the microscopic view of the macroscopic physical phenomena. Since the quantum physics was proposed in early 20th century, physicists study materials in a novel way: particles could have wave-like identities; while waves could also have particle-like identities. For examples, electron, one of the fundamental particles, is intuitively considered as particle. However, a electron could "tunnel through" a potential wall, with which the classic physics could not explain. Quantum mechanics successively describe the phenomena of "penetrating through a energy barrier" effect by considering the electron as a particle wave. This effect is the fundamental background of the Nobel Prize (year 1986) work - the invention of scanning tunneling microscope (STM), which is one of the state-of-the-art research tools used intensively nowadays. Another example is the atom/ion vibration modes in condensed materials. The vibration modes are intuitively considered as waves propagating heat through the interior of materials. However, this picture is failed when Albert Einstein tried to explain the heat capacity of a material with Einstein model, which treats the solid as many individual, non-interacting quantum harmonic oscillators. This issue was solved by Peter Debye who treat the vibrations as "phonons" (Debye model), which are, in concept, particles, or quasi-particles.
In short, to fully understand the physical properties of materials, one has to understand both sides of the identities - wave-like and particle-like behaviors. With the fact that the wave-like properties are easier to be described in momentum space; while the particle-like properties are easier to be understood in real space, scientists use tools probing physical properties either in momentum space (reciprocal space) and in real space. In our lab, as now focusing on studying electronic properties, we use STM as the tool for real space studies and angle-resolved photoemission spectroscopy (ARPES) for momentum space studies. By doing so, we could gain a thorough picture of the electronic properties of the materials of interest.
![]() |
![]() |
Real Space | Momentum Space |
The Material Properties in Low Dimensional Systems
Dimensionality is very important and could alter the physical properties of the materials dramatically. Examples could be found in many nano-scale materials, which exhibit distinct properties from their bulk counterparts. Bulk materials are considered as three-dimensional (3D) materials; surfaces and interfaces are 2D environments; nanowires are 1D materials; while nano-dots are 0D materials. The criteria for the reduced dimensionality, the physical length scale has to be reduced to the length scale of the wavefunctions of the electrons, phonons or other quasiparticles in that particular materials. For 2D materials, such as surfaces and interfaces, the broken translational symmetry, spatial confinement and chemical engineering would be the major degree of freedom responsible for the exotic physical properties. Our lab is devoted to study these 2D physical properties in real and in reciprocal space, in order to gain a full understanding of the underlying fundamental physics, which controls the macroscopic behaviors.
![]() |
Novel Physics at Interfaces and Surfaces |
Research Interests
Currently, here are major research directions that we focus on: (1) utilizing the developed technique of cross-sectional scanning tunneling microscopy and spectroscopy (XSTM/S) for non-cleavable materials on all-oxide electronic devices; (2) next generation solar cell devices; (3) probing magnetic skyrmions; (4) topological and quantum materials; (5) high entropy materials; (6) developing new capabilities with STM; and (7) synthesizing novel and/or high value materials.
Complex Oxides Probed by STM/S
We have successfully developed a controllable way to fracture complex oxide hetero-epitaxial thin film and probed with XSTM/S, which revealed the band alignment between two different complex oxide materials. Complex oxide materials are considered to have great potential for developing novel electronic devices due to its great variety of functionalities. Many of the functionalities could be engineered at the interfaces of the complex oxides, the understanding of the interfaces are essential for the applications. However, since complex oxides, unlike traditional semiconductors, have strong correlated and coupled environment for electrons, spins, lattices and phonon modes, the validity of the conventional semiconductor physics (based on assumption of weak correlation/coupling) on complex oxides are not established. We will focus on probing the interface band interaction of complex oxide in device form while it's on operating condition. Systematic study of the band interaction could pave the path to understand the engineer of the complex oxide electronic devices.
Next Generation Solar Cell Materials Probed by STM/S
Nowadays, energy generation is heavily depended on the fossil fuels. The development of renewable energy sources is essential not only because the goal of reducing the impact on the climate change, but also the goal of replacing the limited fossil fuel resources. Solar energy is one of the renewable energy sources. Cheap and efficient solar cells are the keys to boost the harvesting of the solar energy. Among the different types of solar cells, organic photovoltaic cells (OPVC), quantum-dot sensitized solar cells (QDSSC), and organometallic halide perovskites (OHP) are the promising candidates of next generation solar cell techniques. Despite the intensive study on these devices, the band interaction/relationship at the interfaces of the electron donor/acceptor in OPVC and/or of the sensitizer/photoanode in QDSSC, and nano-scale electronic domains in OHPs are relatively unexplored. In our lab, we focus on extending the developed XSTM/S technique to probe the interfaces of those solar cell devices, in order to reveal the band interaction/relationship in device form under operating conditions. This information could provide the crucial information of further understand the solar cells microscopically and would help to point out the direction of improving the efficiency.
Magnetic Skyrmion Probed by Spin-polarized STM/S
Currently, we are developing the spin-polarized STM (SPSTM) capability, aiming to probe the magnetic skyrmions in various magnetic materials. Magnetic skyrmions are topologically protected magnetic states with specific spin textures. The existing magnetic skyrmions are mainly done with neutron scatter and lorentz TEM techniques. Neutron scattering can only study magnetic skymion lattices (ordered magnetic skyrmions); while the lorentz TEM can study both ordered and randomly distributed magnetic skyrmions. The use of SPSTM could also study both types of skyrmions with an extra capability of providing electronic density of states (DOS) information associated with the skyrmions. EuO1-x is the current focus in our lab. The first step in our research group is to confirm the existence of the magnetic skyrmion in EuO1-x. In addition, we recently started to study CrBr3 monolayer flakes and will later move onto the magnetic properties of these van der Waals 2D materials.
Topological and Quantum Materials
We study the quantum states in various quantum materials. The main research direction toward Quantum Materials is aiming for understand novel quantum states fundamentally with potential of being used as qubit (quantum bit). In particular, we are working on studying the topological superconductity in 2M WS2. The mechanism of the superconductivity, the origin of the topology and the critical thickness of this vdW material that can hold superconductivity and the topology. We utilize the combination of STM and ARPES (in collaboration with Lawrence Berkeley National Lab) to answer these questions.The Majorana zero mode found in the topological superconductor is believed to be ideal qubit for topological quantum computing, in which the qubit is more robust against the noise making it more stable.
High Entropy Materials
High entropy materials, including high entropy alloys, high entropy transition metal dichalcogenides (TMDs), and high entropy perovskite oxides, are complex materials systems involving multiple (typically five) elements occupying the same crystalline sites with same probability. These materials exhibit interesting functionalities as well as provide a playground for exploring the randomness on the physical properties. We aim to study the oxidation process of the high entropy alloys, the Anderson localizations in high entropy TMDs, and random magnetic interactions in magnetic high entropy perovskite oxides.
Developing New Capabilities with STM
STM/S is a powerful tool which can probe electronic properties in nm to atomic scale. Currently, our STM system allow us to study temperature dependent phenomena from 4 K to room temperature. There are ways to further expand the capability of the STM. In our lab, we are working on integrate our STM system with external stimuli application to the materials we are interested in. In particular, we are (a) developing STM/S measurements while applying lateral bias to study materials under steady state condition; (b) STM/S measurements with light illumination to study material interactions with light; and (c) STM measurements with magnetic field applications.
High Value Carbon-based Materials from Wyoming Coals
Coal, an energy source used mostly by combustion to produce electricity, is one of the major natural resources and economic sectors in the state of Wyoming. It contains mostly carbon-based small molecules or materials that have great potential to be used as other type of high value materials. To diversity Wyoming economy, we are working on seeking strategies to extract and characterize the high value carbon-based materials out from the Wyoming coals.
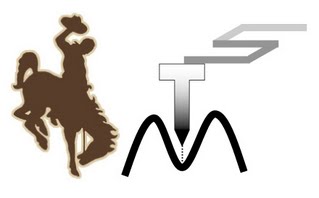